The Monstrous Maw
André Hoelz
California Institute of Technology
Published June 28, 2023
André Hoelz was visiting The Rockefeller University in New York on a graduate school scouting trip when he heard an unforgettable talk. The lecture centered on an immense molecular complex essential to the flow of genetic information in plants, animals and people.
Called the nuclear pore complex, it speckles the nucleus within cells like craters on the moon. Each complex pierces the double membrane that shelters chromosomes from the harsh cellular environment. In the genetic flow of life, DNA makes RNA inside the nucleus. Then, through the nuclear pore complexes, RNA exits into the main cellular compartment to make protein.
The pore complexes are more than genetic escape hatches. They manage two-way traffic between the nucleus and the main compartment, or cytoplasm, of the cell. For example, they also provide restricted access to incoming signals, such as to transcribe a gene for a protein the cell needs.
In the tiny world of the cell, the pore complex ranks as one the largest molecular assemblies. It has been called a "monstrous maw," a gaping portal more than 100 nanometers across. About 1,000 individual proteins create three ring structures, one inner ring encircling the central transport channel and two outer rings anchored on each side of the nuclear envelope.
But it wasn't the shape or size of the complex that fascinated Hoelz. It was the tentacles, a mesh of fluttering protein filaments thought to dictate what molecules may pass through. "It was absolutely amazing," he says.
So it is fitting that two decades later, those fluttering filaments were some of the last parts to come into focus when the Hoelz lab and others published a handful of papers that essentially completed a composite structure of the pore complex (Science 2022).
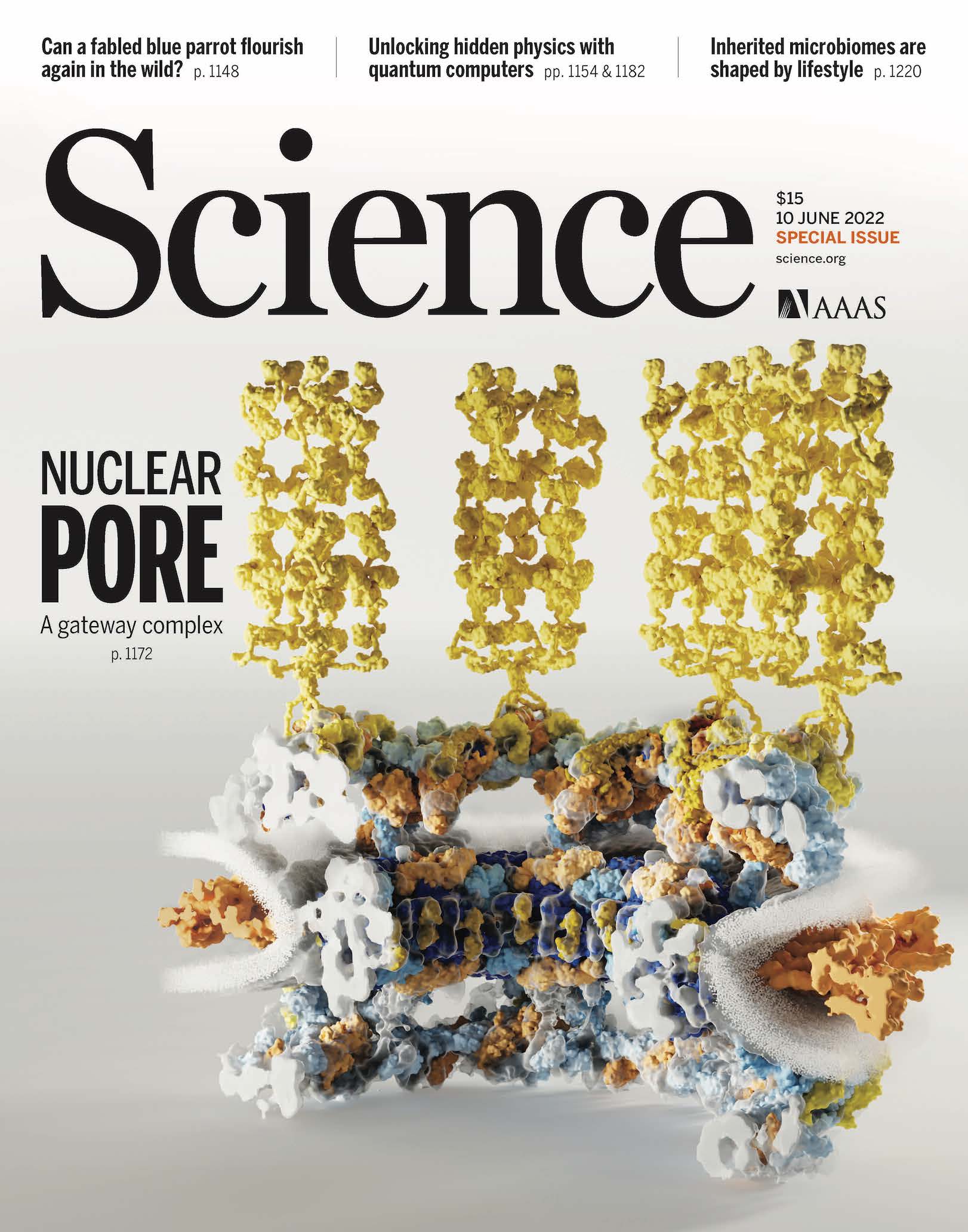
"In some ways, it became really clear that the thing was done when the Science editors basically said, 'We're going to make a special issue,'" says Hoelz, who runs a lab at California Institute of Technology in Pasadena.
The static reference structure may be done, but Hoelz is not finished with the nuclear pore complex. For one, there are some blind spots to elucidate and a few details to confirm experimentally. As to the next big lab projects, he is taking a cue from others who resolved major structures, such as the ribosome and the potassium channel, and then pivoted to studies of their functions.
"We have more value by actually applying the things that we have learned and trying to solve the riddles of the pore and the nucleocytoplasmic transport," Hoelz says. "And that's also where my heart is. I really want to understand how this machine works." And he wants to see how the structure moves.
Still, that leaves a lot of possibilities. "The dynamics of how the pore exports RNA is a really, really big one," Hoelz says. "The pore is also targeted by a whole bunch of [virus] virulence factors. SARS-CoV2 also has a virulence factor that very effectively can shut down the pore."
The cytoplasmic face of the complex, with those fluttering filaments, may hold the key to understanding and perhaps treating some intractable diseases. Cancers with mutations in nuclear pore complex proteins (nucleoporins) have poor prognoses, he says. The Hoelz lab has collaborations in progress to explore the implications of nucleoporin mutations in amyotrophic lateral sclerosis (ALS) and other neurodegenerative diseases.
Only three dozen distinct nucleoporins assemble in repeat symmetry to create a pore complex. The complexes don't appear to renew efficiently in cells that don't divide, such as neurons. Hoelz speculates that some genetic variations work well enough for 20 or 30 years, but maybe not for the lifetime of a neuron.
Other intriguing questions include how the pore is assembled in the nuclear envelope, how it is taken out or recycled when cells divide, and what factors have a hand in all the pore action.
"It's a good time for people to come up with ideas, start them, and then wrap it up and take it with them," he says, referring to young scientists who may want to join his group and apply the lab's approach to related molecular machinery.
Hoelz grew up in Germany to an optician and ophthalmologist (father) and economist and businesswoman (mother). The family settled in the southern town of Schwäbisch Hall, known for its gorgeous medieval architecture and also for being the headquarters of a bank that specializes in home building savings accounts, akin to college funds, started at a child's birth, and known countrywide for its catchy advertising jingle. Hoelz loves to cook, and for his lab he sometimes cooks a specialty from the nearby Alsace region, tarte flambée, a thin pizza-like flatbread traditionally topped with fromage blanc, onions and bacon.
He went to a high school that specialized in economics, ranging from world economies to bookkeeping. He had a second emphasis on chemistry, which he found unexpectedly intuitive. "I was not bad in school," he says, "but this was something that was especially really easy for me."
His affinity for chemistry was nurtured by a teacher who had a PhD in inorganic chemistry, the happy result of a national incentive program launched during a shortage of science teachers in Germany. He also had a good biology teacher. Together, they inspired him to become a biochemist.
At the Albert Ludwig University of Freiburg, Hoelz completed a Vodiplom in chemistry and a Diplom in chemistry and biochemistry, the equivalent of bachelor's and master's degrees. He spent more time in the lab than in lectures. "You learn things in the in the lab that are very different from the textbook," he says. “And that’s what I tell the undergrads: Don’t just learn science from the books, go to the labs and actually see it, because then you don’t have to memorize it, you will just intuitively gain that knowledge.”
For graduate school, he applied only to Rockefeller. For his doctoral work, Hoelz joined the lab of John Kuriyan, where he worked on the structure of a protein kinase that regulates calcium signaling and is important for learning, memory and cardiac function (Molecular Cell, 2003). Kuriyan left to join the faculty at University of California, Berkeley, but remained his official thesis advisor. Hoelz stayed, unwilling to perturb the crystals he finally had grown.
He finished his PhD in the labs of Thomas Sakmar and Nobel Laureate Günter Blobel, who had given the memorable talk on the nuclear pore complex on his original visit. When Hoelz began to look for postdoc positions, Blobel invited him to the beautiful Rockefeller garden for a talk. Blobel noted Hoelz's lingering enthusiasm for the pore complex structure and suggested he stick around to work on it.
Hoelz was skeptical. How could one person have any significant impact? The answer from Blobel: Why don't you start your own lab in my lab? Hoelz accepted the offer, appreciative of the close-knit Rockefeller research culture and the opportunity to work with some of the best cell biologists in the world.
There he stayed for the next six years. When it came time to apply for a job, he held out for a position that came with resources to continue work on the pore. In 2010, he started his lab at Caltech. The perks included a molecular observatory that supported x-ray crystallography with a beam line at the Stanford Synchrotron Radiation Laboratory (SSRL). The beam line, in turn, featured a new Pilatus detector, the first one installed in the United States, a technology he calls "transformative."
At Rockefeller, Hoelz took a jigsaw approach to the formidable challenge. Rather than trying to image the elaborate assembly at once, he and his group decided to tackle the crystal structure of each of its three dozen protein subunits and piece them together. With that strategy, they published some of the first structures of how key nucleoporin domains fit together and interact with their partners. These provided hints that the larger architecture involved layered structures and concentric cylinders (Cell, 2008), as well as a prediction that the outer rings were comprised of a head-to-tail arrangement of a major component called the Y complex (PNAS, 2009).
"At Caltech, we expanded this massively, by trying to reconstitute the entire symmetric core complexes," he says. "If you want to get the entire architecture of the pore, then the questions are not individual projects. It's not just one structure that will give you this answer, you need to have a collection of structures."
They developed purification protocols for the 35 unique proteins of the pore and solved the key interactions needed for them to come together. They primarily use bacteria cultures to grow nucleoporins from various fungal species and the human counterparts.
Hoelz designed teams of graduate students and postdocs that could answer a major question within a reasonable amount of time. Parts of the problem were divided at the beginning, with individual people taking lead on individual structures. But if one part turned out to be much more complicated, the entire team would come together to tackle the bottleneck.
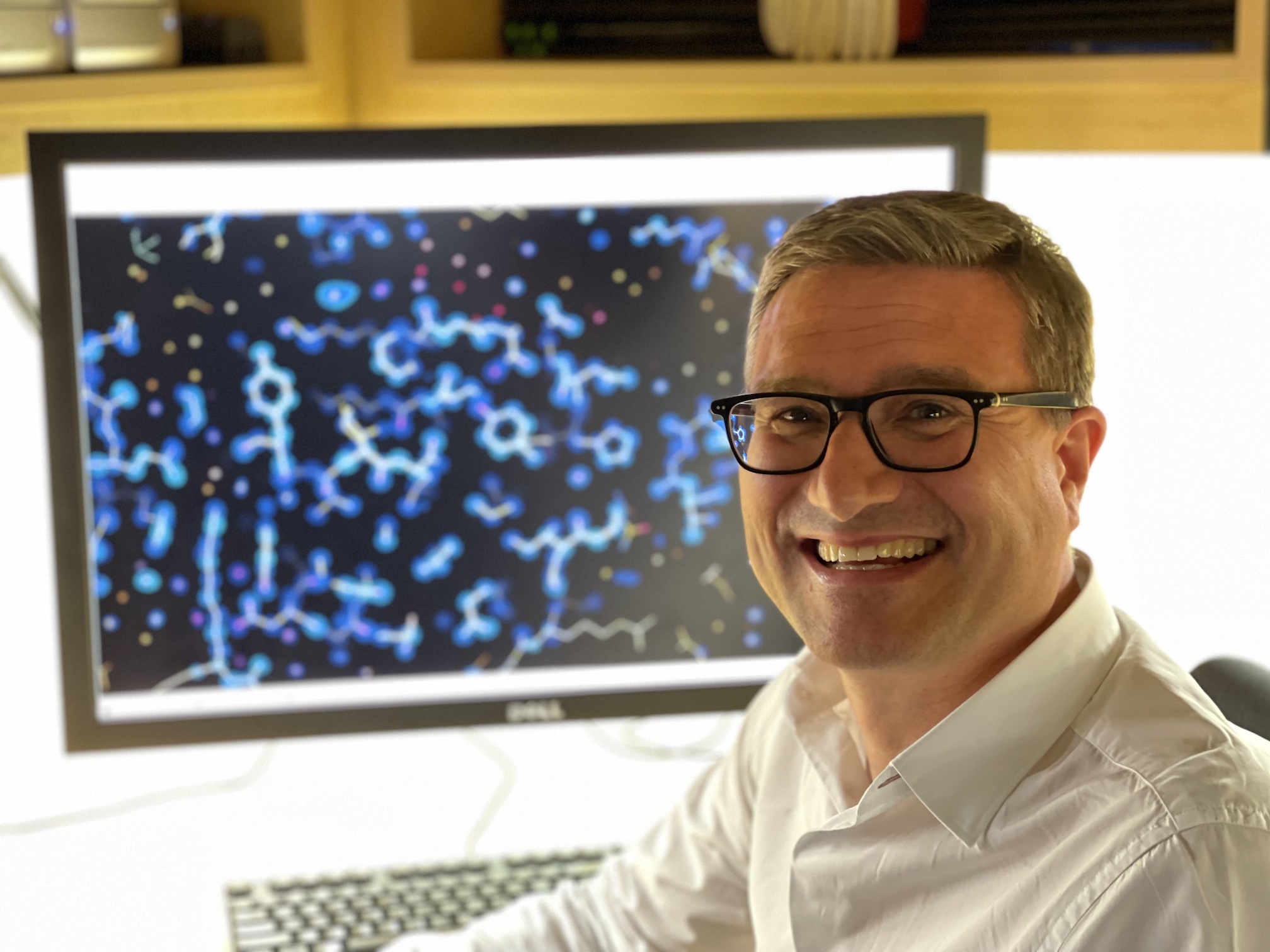
""And I think that sort of approach to science is the secret of our success," he says. Another major component for the full nuclear pore complex structure was a trusted relationship with a German lab using complementary methods, which soon proved to be a powerful combination to elucidate the large machinery.
While the Hoelz lab was assembling structures from the inside out, Martin Beck, now a director at the Max Planck Institute of Biophysics in Frankfurt, was working from the outside in, applying cryo-electron tomography (cryo-ET) to visualize intact nuclear pore complexes isolated from human nuclear membrane at higher and higher resolution.
Publication by publication, the advances from each lab coincided. For example, Beck's group published a 32-angstrom resolution cryo-ET structure of the human pore that showed two rings on either side of the nuclear envelope (Cell 2013), confirming the earlier prediction from the Hoelz lab that Y complexes are arranged in head-to-tail rings.
Meanwhile, the Hoelz group determined the atomic structure of the entire Y complex. They found it was the right size and shape to dock into the Beck lab’s cryo-ET structure of a nuclear pore complex from a human cell (Science, 2015).
"This was a very exciting moment for us,” says Hoelz. “The docking bridged for the first time high and low resolution structural methods and established a near-atomic structure for the pore’s outer rings that predicted where the other nucleoporins were located.”
"Now we had all the ingredients to solve the rest. We just needed to solve all of the remaining nucleoporin structures and figure out how they fit together," Hoelz says. “If you have a box of puzzle pieces, and you start putting them together in the frame, you know that with the same approach, you're gonna get the entire thing done."
The EM map enabled the Hoelz lab to fit more detailed structures of the pore complex components into place. Hoelz and Beck published landmark back-to-back papers using 23 angstrom cryo-ET data and several dozen crystal structures focusing on the entire symmetric core composed of the inner ring and outer rings (Science, 2016).
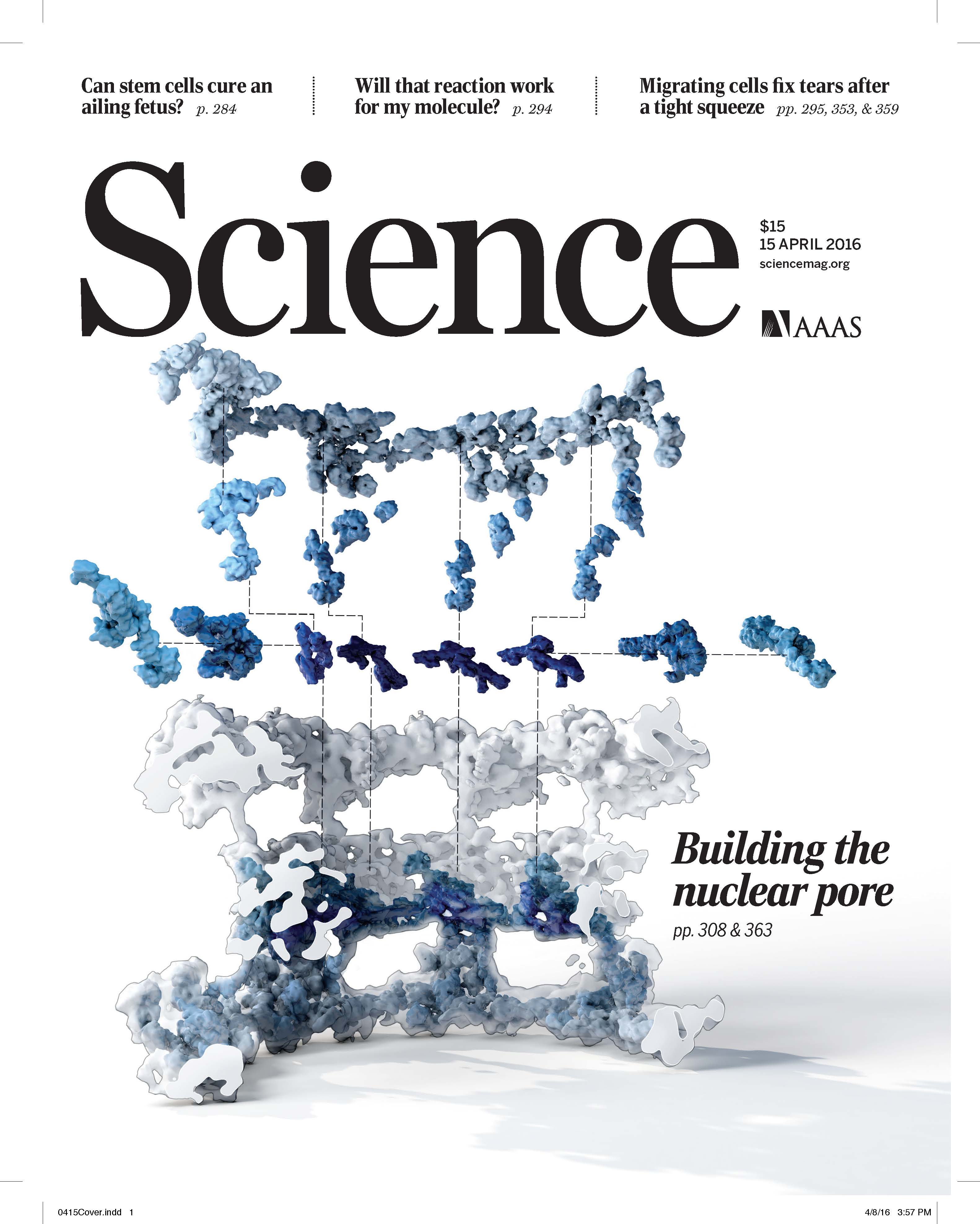
The last major steps were dialing in the linker proteins that connected inner and outer rings and held the inner ring together, as well as getting the details of the fluttering filaments that guard the gateway on the cytoplasmic side. In March 2020, when the COVID-19 pandemic hit, the labs had just exchanged unpublished data with the intention of publishing their independent analyses as companion papers. Beck was pursuing a 12-angstrom electron tomography map.
The Hoelz and Beck groups published the full structures in a special issue (Science, 2022). Two papers from Hoelz group contain four dozen crystal structures. One paper reports how linker proteins connecting the inner and outer rings in different ways act like rubber bands and allow the central transport channel to dilate like an iris of an eye. Another paper illuminated how the 10 different filament nucleoporins—which carry out the terminal steps of mRNA export (and are associated with human diseases)—are arranged and anchored on the side that faces the cytoplasm.
Hoelz expects the reference structure of the nuclear pore complex will trigger an avalanche of new structural data about the dynamics and how different pieces are associated with diseases.
"I really wish he could have seen it," says Hoelz, referring to Blobel, who died in 2018 at age 81. "I can tell you this: I followed his advice to work on something fundamental and major that is solvable in my lifetime."
-Carol Cruzan Morton