Floppy Physics
Eva Nogales
University of California, Berkeley
Published November 30, 2022
At the heart of human cells, tiny protein tubes radiate out in all directions. Anchored near the nucleus, they help cells hold their shape, organize internal components, and in dividing cells, tug apart the duplicated chromosomes. Called microtubules, the filaments constantly grow and shrink, fall apart and put themselves back together.
In the University of California, Berkeley, lab of Eva Nogales, microtubules have shaped a more enduring thread of discovery.
"They have this amazing behavior called dynamic instability—that is, a nonequilibrium state where they can be growing, and then suddenly they start depolymerizing," she says. "It's a stochastic process that in the cell is highly regulated but that you can reproduce in the test tube."
Finely tuned dynamic instability is so important to the essential functions of microtubules that one class of cancer-fighting compounds—the Taxol family—interrupts cell division by stabilizing microtubules, foiling the final fall-apart stage necessary to separate the daughter cells.
Microtubules start as dimers of the protein tubulin that assemble themselves into strands that come together in straw-like tubes. Nogales began studying them during her PhD. But it was 10 years later—as a professor, senior faculty scientist at Lawrence Berkeley National Lab (LBNL) and Howard Hughes Medical Institute (HHMI) investigator—when her team made the technical breakthrough and biological insight that laid the foundation for the lab to pursue applications as diverse as neurological disorders.
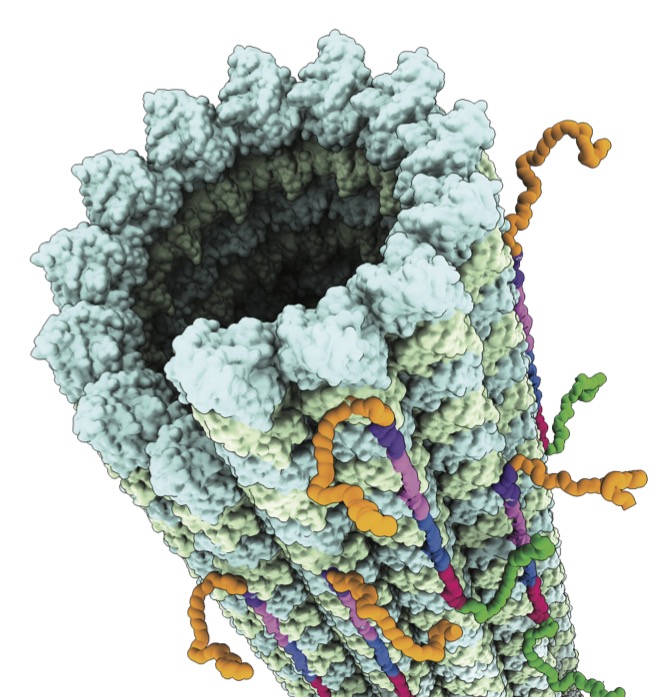
The team had used new methodology to attain the highest resolution at the time for tubulin dimers in association with a microtubule (Cell, 2014). Importantly, the nearly atomic-level peek both suggested a mechanism that makes microtubules fall apart and explained how Taxol prevents the depolymerization.
"For tubulin to associate with a microtubule, it has to be loaded with GTP," Nogales explains. "But after it associates with a microtubule, the GTP gets hydrolyzed to GDP, and in this state tubulin is under tension, and that tension can build, and eventually the microtubule bursts"—except in the presence of taxol, which supports tubulin in a GTP-like conformation, they found.
Over time, the Nogales lab has refined their Taxol model and investigated other microtubule proteins. The protein tau, for example, helps build and maintain microtubules in axons. When it detaches from microtubules, tau can form abnormal tangles implicated in Alzheimer's and other neurological diseases. The structure of the tangles was known, but Nogales and her team determined the detailed structure of the tau protein bound to microtubules in its physiologically healthy state (Science, 2018).
Nogales grew up in Spain in a small town north of Madrid. The 40-year dictatorship of Francisco Franco ended when she turned 10. Her father, from a family of farmers, was a shepherd, and her mother did embroidery, part of an impoverished generation that came of age when only the very rich attended high school and college. Her parents wanted their children to have everything they didn't and saw education as the primary means.
"So we may not have had money for other things, but we had money for books," she says. "Everything that has to do with the school we would get, because that was the most important thing."
The country quickly transitioned to democracy, with people voting for the first time. But a repressive atmosphere for women lingered. Nogales attended a small high school serving local villages. One of the few sanctioned professions for women outside the home was teaching. Three of Nogales' favorite high school teachers were women who taught mathematics, physics, and biology and who, she realized in hindsight, served as sustained role models.
"They were three women with super strong personalities…very passionate, very, very capable," she says. "And I think I am who I am, because I had that reference."
In fact, when she published her first cover paper in Nature, Nogales contacted them and arranged a reunion in Spain. "They're long retired now, but they're still very inspiring," she says. "One is still writing physics books. The other one is super active in charities, and the other one still works with the high school students to help with scientific writing."
She was one of three students at the top of her graduating class, one of whom went into medicine and another whose father didn't allow her to go on to college. Nogales's brother studied engineering, perhaps the most elite occupation in Spain at the time. Naturally curious, she chose physics and earned her BS at Universidad Autónoma de Madrid.
"What I appreciate most is the sense of awe—at nature or a novel or many other things," she says. "I wanted to not only read about nature, but actually be the one participating in the discovery."
She moved to the United Kingdom for her PhD, an opportunity born of meeting the director of the British synchrotron at a summer conference and a Spanish fellowship designed to prepare scientists for the European Synchroton Radiation Facility (ESRF) under construction in Grenoble, France. She switched to biophysics, taking her first look at tubulin polymers with advisor Joan Bordas at Daresbury Laboratory.
She started with small angle X-ray scattering. But she found the information "a little too indirect" and sought tutelage from Dick Wade, on sabbatical from Grenoble, to help prepare frozen solutions of microtubules for cryo-electron microscopy (cryo-EM) visualization. The technique was in its infancy, but other scientists were already making advances that would culminate in the 2017 Nobel Prize in Chemistry.
In 1993, she finished her PhD and moved to California, where her boyfriend, soon husband, was hired as head of experimental systems at the new Advanced Light Source at LBNL. At the time, X-ray crystals dominated structural biology, but Nogales saw a better future for herself in cryo-EM, even though there were only a few, well-behaved proteins that could be studied that way.
She landed in the LBNL lab of Kenneth Downing, who offered her a postdoctoral position based on her experience with tubulin and cryo-EM. Five years later, she was the lead author on a paper describing the first structure of tubulin, including the location of the Taxol binding site (Nature, 1998). She accepted a job offer at UC Berkeley and two years later also became a HHMI investigator.
Since its early days, her lab's research has run in parallel with the capabilities of cryo-EM. They have studied some systems for many years, the experimental cycle often starting with a structure at 40 angstrom resolution, then getting 30 resolution, and ultimately generating atomic models.
"We were pushing the methodology for our particular samples," Nogales says. "We are not a methodology-developing lab per se, but when we are trying to solve a certain biological problem, if the tools are not there, we will develop them," such as to address the pseudo-helical assembly of microtubules.
In another milestone, graduate students in her lab solved the structure of the human transcription factor TFIID, a huge and floppy agglomeration of more than a dozen distinct proteins (Science, 2018). The complex finds the sequences in the genome where protein-making genes start and then confirms it is, in fact, in the correct spot. Then it opens up to recruit dozens of other proteins that then start ratcheting along the gene, using the DNA sequence as a template to make messenger RNA. The structure was the culmination of a 20-year collaboration between the labs of Nogales and Berkeley HHMI colleague Robert Tijan.
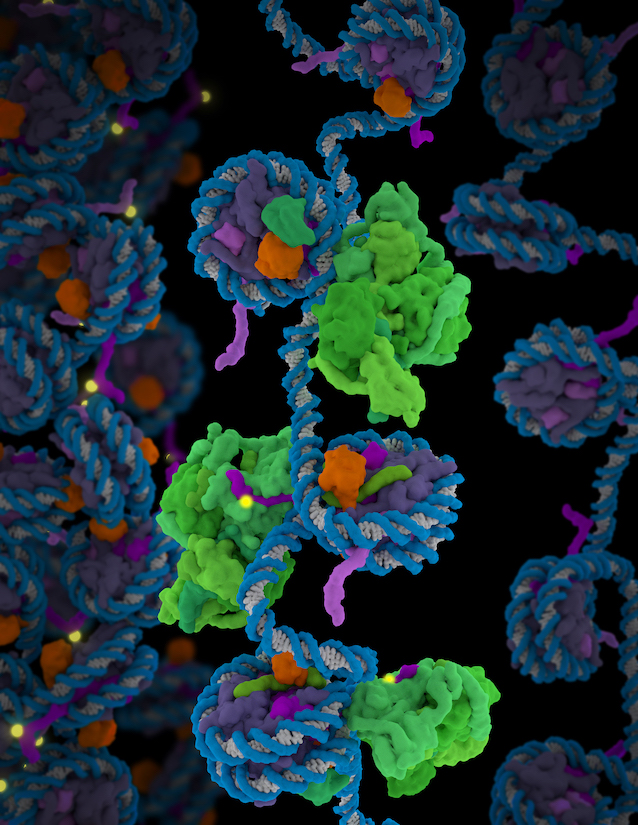
"It is flexible, incredibly flexible," Nogales says. "It has three major lobes, and one lobe moves by 150 angstrom. It made it really difficult for us to get a structure. We were describing it at different stages as time went along, until the new detector technology and things like RELION came along, where you could separate states and do the different pieces separately."
The team built the model of all the components and related the motion of the complex to the DNA binding, including the release of a key protein in nucleation after TFIID has verified its genomic touchdown site.
In the lab and life, the human factor is as important as the technical capabilities, Nogales says. "When it comes to the day to day, science is grinding," she says. "You want to be surrounded by people that may be able to help you technically, but also support you emotionally, and among whom you just feel part of a team. Because alone we cannot achieve much. It's just too hard. We will be disappointed and frustrated too soon. Sometimes you need someone that picks you up and gives you new energy. And if you have enough of these warm bodies around, they can transmit such energy from one to another. Sometimes one of them will be in an emotional valley, at a point where it may feel like it is the end of the road, but another one will come and bring them up. Another day the roles will be reversed."
During the pandemic's first two years, Nogales picked up the pace of her reading, consuming mostly fiction by contemporary women in English and Spanish. But as in-person meetings resume, she is looking forward to her self-appointed extracurricular duty to get the dancing started after the closing banquet of the conference.
-Carol Cruzan Morton