Changing channels
Daniel Minor Jr.
University of California, San Francisco
Published September 27, 2019
Kissing a frog might not spark a fairy tale ending, but learning how frogs survive some of nature’s most deadly poisons has opened a new chapter in the laboratory of Daniel Minor Jr.
The potent neurotoxin saxitoxin, for example, can come from algae blooms in fresh, brackish, and oceanic waters, such as red tides, which seem to be on the increase from climate change and other factors. The toxin accumulates in clams, oysters, and mussels. If people eat contaminated shellfish, they can die within hours. Saxitoxin has a lethal dose a thousand times smaller than deadly amounts of cyanide or sarin. It is the only marine toxin classified as a chemical weapon.
Importantly for Minor’s research, saxitoxin is believed to exert its deadly effects mainly by blocking sodium channels, paralyzing the muscles needed to breathe.
Bullfrogs, on the other hand, aren’t fazed by the toxin, thanks to a protein they make called saxiphilin. In June 2019, Minor’s University of California, San Francisco, team and their Stanford University collaborators revealed the molecular architecture that allows saxiphilin to sop up the toxin.
The paper in Science Advances describes the molecular shapes and dynamics of saxiphilin alone and bound to saxitoxin. It is the first structural characterization of a “molecular sponge” for a toxin, Minor and his co-authors write. Interestingly, the way the toxin binds to saxiphilin to save the frog is similar to the way the toxin blocks ion channels to poison people.
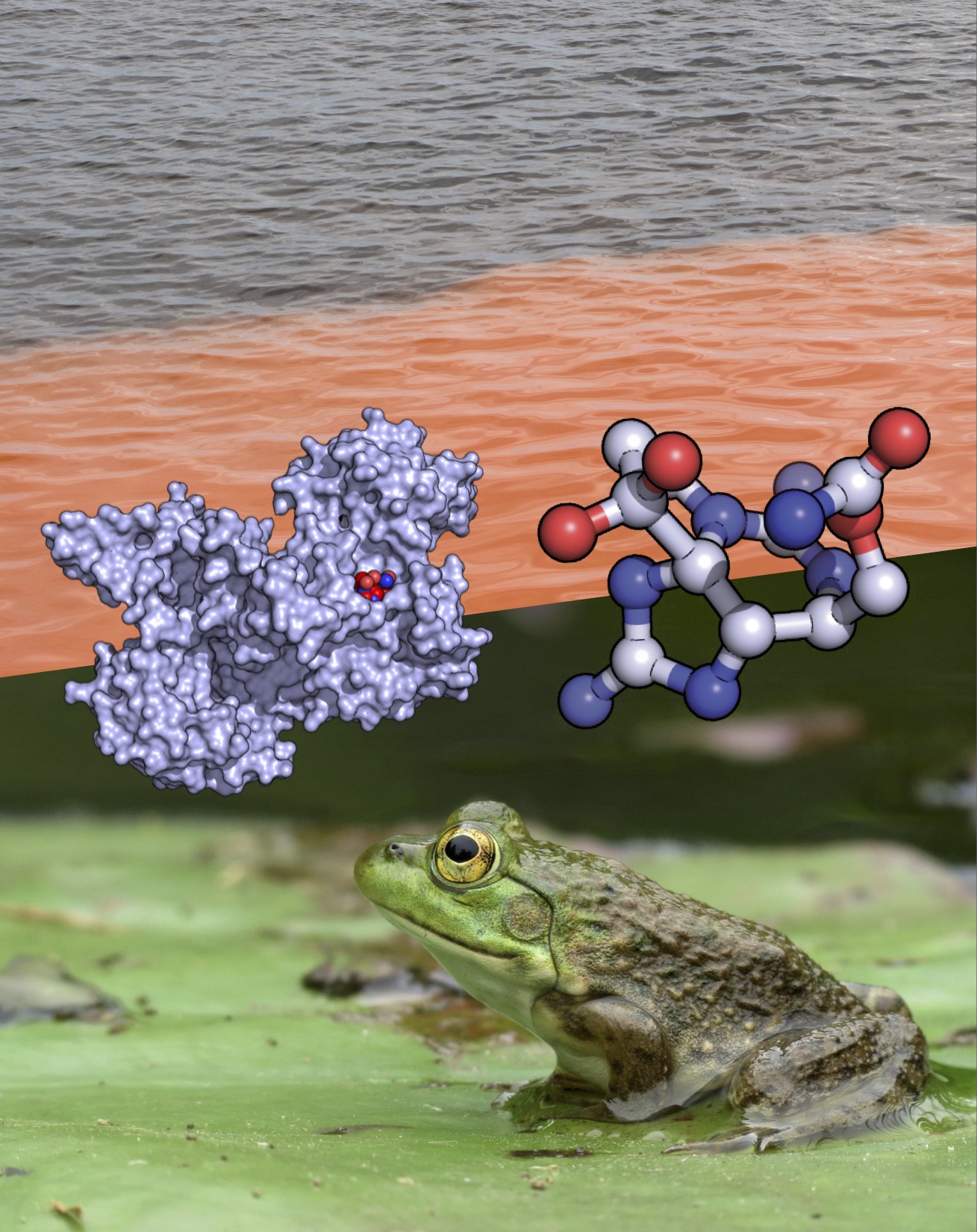
Minor’s research group is interested in ion channels. The tiny pores carry electrical currents into cells that prompt thoughts, sensations, and actions. One system studied in the lab, voltage-gated sodium channels, could lead to new therapeutics for cardiac arrhythmias, movement disorders, pain, migraine, and epilepsy.
But there is another potentially lifesaving angle that Minor had not considered until recently: Preventing and treating poisoning caused by deadly neurotoxins.
“We’re really excited by this auto-resistance mechanism—how an organism deals with a toxic environment and how it survives,” says Minor. His laboratory collection of frogs, toxins, and questions has since expanded. “Poison dart frogs have all these toxins that target sodium channels. Why don’t the frogs kill themselves?”
Minor wants to understand more about how nature has solved the problem of handling these toxins. He also wants to learn how the defensive frog compounds modify sodium channels and use that information to develop new ways to control channel activities in neurons.
The answers have additional public health and commercial implications. The findings may lead to better sensors to screen for toxins in shellfish destined for human consumption. Further work could lead to an antidote for poisoning by toxins in shellfish or chemical weapons.
Having a lab full of frogs wasn’t exactly something Minor had planned, but in high school he was interested in science. In fact, he secretly set his sights on the storied Laboratory for Molecule Biology (LMB) in Cambridge, UK, after reading Freeman Judson’s Eighth Day of Creation about cracking the DNA code.
“To become a scientist was not a normal thing,” says Minor, about his working-class Northeast Pennsylvania hometown. Good students might strive to become doctors or lawyers. His family supported his decision anyway. “But it’s really weird—when I was growing up, our yard faced the yard of another family, and their son also became a scientist. Both [sets of] parents are not sure what happened on this block.”
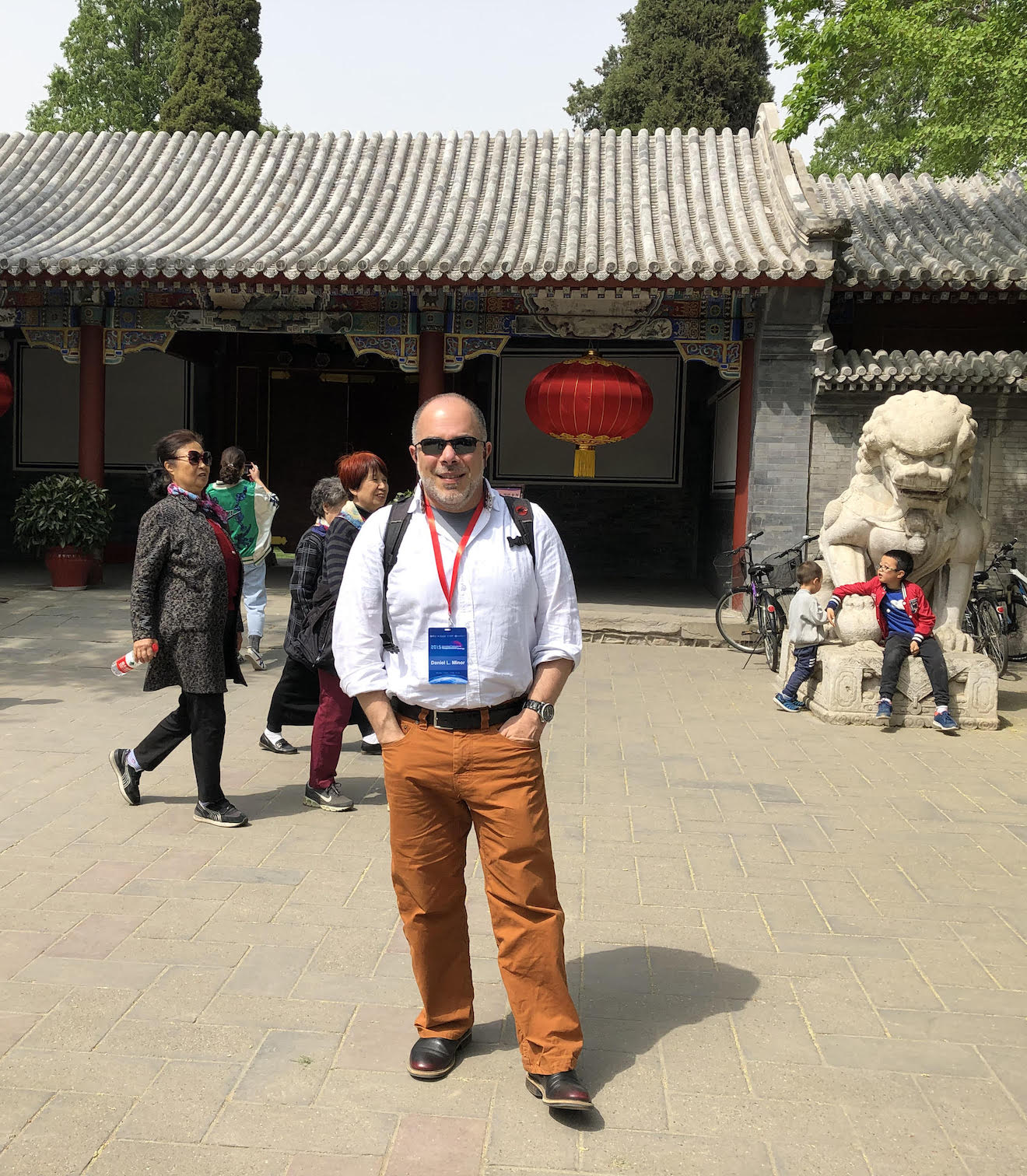
Minor grew up in a family where no one had gone to college and few finished high school. Both grandfathers had worked in the coal mines. The next generation worked in some form of light manufacturing, including Minor’s father, who rose from worker to manager of a factory that made crayons and tempera paints. At age 14, Minor went to work part-time in the factory, loading trucks and mixing pigments with chalky calcium carbonate powder.
Evenings, Minor trained as weightlifter at the local YMCA. He competed in tournaments at the Holy Savior Weight Lifting Club in the basement of a Catholic church near Philadelphia. He specialized in the snatch and the clean and jerk and at age 16 was state powerlifting champion.
As a work-study undergraduate at University of Pennsylvania, Minor avoided slinging hamburgers by finagling jobs in various research labs focusing on proteins, cell biology, and nuclear magnetic resonance. As a graduate student, he joined Peter Kim’s lab at Massachusetts Institute of Technology.
There, he published three Nature papers, the most important showing how much context matters to the way proteins fold. Depending on its amino acid neighbors, for example, the same 11-residue “chameleon sequence” he designed could fold into a beta sheet or a helix. Memorably, Nobel Laureate Max Perutz noted Minor’s discovery in a perspective piece. “That was awesome,” he says.
For his postdoctoral fellowship, Minor was interested in brain and membrane proteins, especially ion channels, a field driven at the time by mutation studies. He wanted to add a structural dimension. That meant either going to a structural lab and being the lone person working on the problem, or joining an ion channel group and being the lone structural person.
In a flurry of indecision, Minor cycled through three labs. He started in Boston and followed a soon-to-be-ex girlfriend to Cambridge, UK. There, he fulfilled a childhood dream as a postdoc at the LMB working with Nigel Unwin for a short period. He reset his postgraduate research at UCSF, where the wife-husband team of Lily and Yuh-Nung Jan were working at the forefront of cloning channels.
“Two things amazed me about Lily,” he says, “She always made time for me, and she mostly thought my ideas were good.” She encouraged Minor to develop a bigger picture perspective, a legacy he wants to pass on to others. There, he built an ion channel skill set portfolio from function to structure and back.
When he moved to San Francisco, Minor dropped his LMB project, a modular approach to the nicotinic acetylcholine receptor. Meanwhile, a talented postdoc who shared a passion for acetylcholine receptors was working in an Amsterdam lab and had found another way to solve a structure of the receptor extracellular domain. They met at a structural biology meeting, bonded over their interest in the nicotinic receptor, and eventually married. Their wedding invitation featured the structure she solved.
“It’s a good lesson,” says Minor, who since age 16 strived to plan ahead thoughtfully and make the right choices. “Here’s an example where my best-laid plans all fell apart, but ended up working out better than I could ever have imagined.”
His good fortune held. He applied for a geneticist job opening in the UCSF Cardiovascular Research Institute. He had worked on yeast genetic selections to study ion channels in the Jan lab, and there are ion channels in the heart. These two factors and a broad view of the search committee led to a job offer. “They just wanted a good scientist working on cardiovascular-related topics,” he says.
Minor began working on ion channels just as other groups had cracked the first ion channel structures, and atomic protein measurements were starting to become relevant to a field that had long relied on mutational and electrophysiology studies. “Their functional relevance took some time to percolate into the field,” he says.
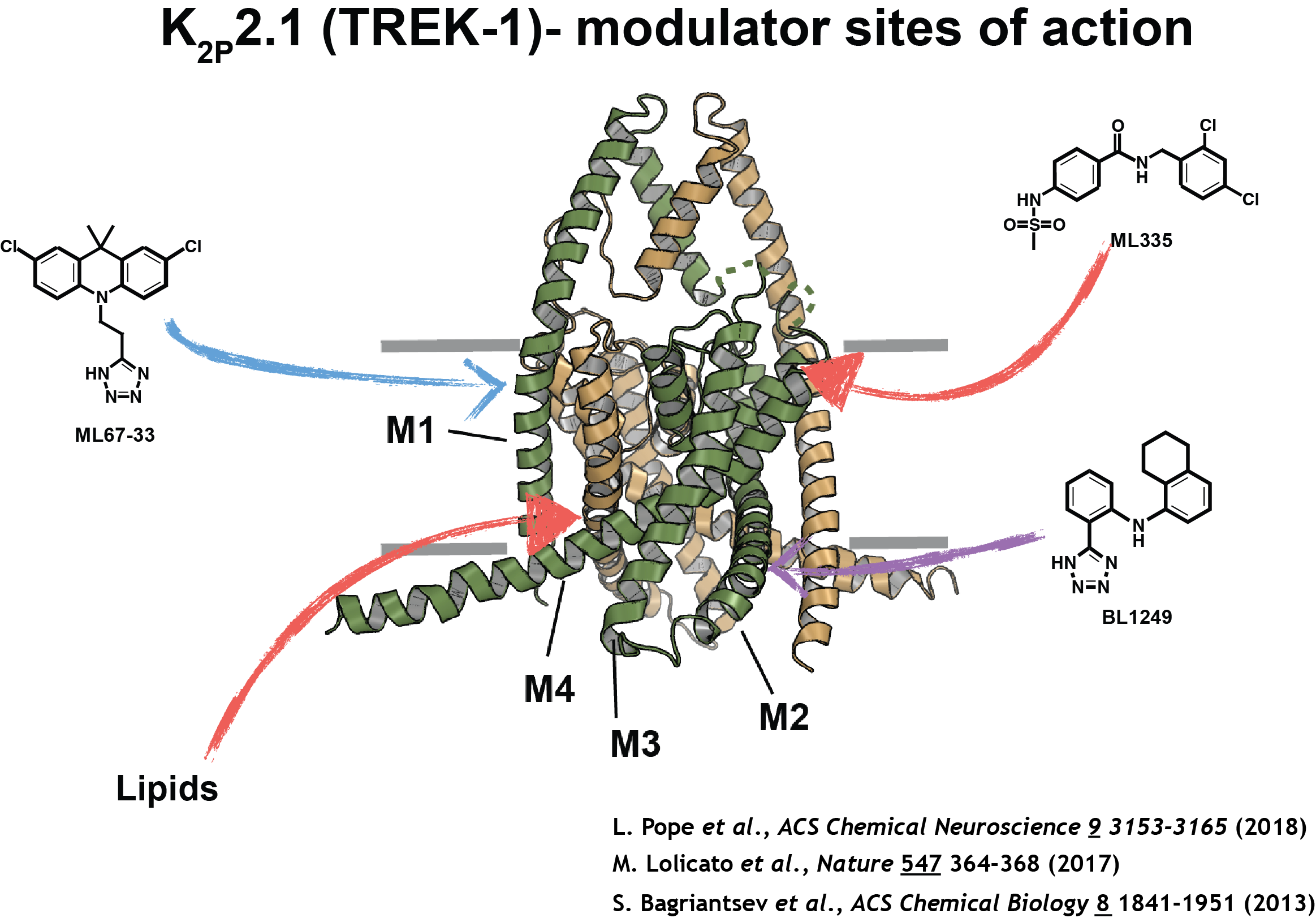
The structure of K2P2.1 (TREK-1) shows modulators and their sites of action, defined structurally (red arrows) or functionally (blue and purple arrows).
His integrated structural approach has shown new ways that protein structure changes affect ion channel activity. For example, a 2017 Nature paper discovered a novel small molecule binding site that props open members of the TREK-1 K2P potassium channel family important for pain, analgesic responses, and mood. “It’s a beautiful example of the need for conformational change to make a binding site,” he says.
Meanwhile, the Minor lab also runs a program to find small molecules that can selectively inhibit ion channels for scientific study and for drug discovery.
The thread that ties the work together is protein chemistry, he says. “We’re trying to understand how proteins work, how they move, how they function, and how we can learn to control their actions.”
-Carol Cruzan Morton
Other tales
-
The Final Phase
George Phillips
Published 31 May 2025
Mind and Muscle
Ryan Hibbs
Published 28 March 2025
-
The Shapes of Energy
Luke Chao
Published 12 December 2024
Predicting Proteins
Jens Meiler
Published 25 November 2024
-
Death Metal
Steven Damo
Published 28 April 2024
Context Matters
Bing Chen
Published 30 January 2024
-
The Crystal Whisperer
Sarah Bowman
Published 29 November 2023
Data in Motion
Nozomi Ando
Published 29 September 2023
-
The Monstrous Maw
André Hoelz
Published 28 June 2023
Second Takes
Andrea Thorn
Published 28 February 2023
-
Radical reactions
Yvain Nicolet
Published 31 January 2023
Floppy Physics
Eva Nogales
Published 30 November 2022
-
Structure of Equity
Jamaine Davis
Published 28 September 2022
Life and Death of a Cell
Evris Gavathiotis
Published 28 July 2022
-
Follow the glow
Kurt Krause
Published 29 April 2022
Resolution solutions
Willy Wriggers
Published 25 February 2022
-
Of enzymes and membranes
Ming Zhou
Published 28 October 2021
Step-by-step
Gabrielle Rudenko
Published 26 September 2021
-
Moving muscle
Montserrat Samso
Published 26 July 2021
Particle catcher
Stefan Raunser
Published 28 June 2021
-
Designer drugs
Ho Leung Ng
Published 25 February 2021
Right place, right time
Ernesto Fuentes
Published 29 January 2021
-
Shape-shifting secrets of membranes
James Hurley
Published 27 November 2020
Enzymatic action
Cynthia Wolberger
Published 28 September 2020
-
Rules of motion
Priyamvada Acharya
Published 31 July 2020
Cosmic Squared
Michael Cianfrocco
Published 27 June 2020
-
Kaps are Cool
Yuh Min Chook
Published 28 April 2020
Spiraling into focus
Carsten Sachse
Published 29 March 2020
-
Seeing cilia
Alan Brown
Published 27 February 2020
For the Love of EM
Guy Schoehn
Published 27 January 2020
-
Protein Puddles
Michael Rosen
Published 16 December 2019
Changing channels
Daniel Minor Jr.
Published 27 September 2019
-
Listening Tips
Marcos Sotomayor
Published 30 July 2019
Beyond Cool
Published 31 May 2019
-
Hao Wu
A Higher Order
Published 30 May 2019
Aye Aye Captain
Alexandre Bonvin
Published 29 April 2019
-
The PARP Family Family
John Pascal
Published 28 February 2019
Frame by frame
Nikolaus Grigorieff
Published 28 January 2019
-
Predicting Success
Bil Clemons
Published 18 December 2018
Curiouser and Curiouser
Ramaswamy Subramanian
Published 27 November 2018
-
Rely on This
Sjors Scheres
Published 26 October 2018
Proteins out of bounds
Gerhard Wagner
Published 27 September 2018
-
Hiding in plain sight
Gaya Amarasinghe
Published 27 July 2018
Jumping Genes
Orsolya Barabas
Published 27 June 2018
-
Data Whisperer
Karolin Luger
Published 30 May 2018
Flipping the Switch
Jacqueline Cherfils
Published 27 April 2018
-
Tooling Around
Andrew Kruse
Published 29 March 2018
Comings and Goings
Tom Rapoport, Ph.D.
Published 23 February 2018
-
Transcriptional Rhythm
Seth Darst
Published 27 January 2018
The Language of Gene Regulation
Daniel Panne
Published 21 November 2017
-
Not Your Average Protein
James Fraser
Published 23 October 2017
Message Received
Sebastien Granier
Published 24 August 2017
-
Resistance is Futile
Celia Schiffer
Published 28 July 2017
Twist of Fate
Leemor Joshua-Tor
Published 28 June 2017
-
Drug Designer
John Buolamwini
Published 30 May 2017
Mathematically Minded
James Holton
Published 28 April 2017
-
Garbage Out
Kay Diederichs
Published 30 March 2017
Fixer Upper
Brandt Eichman
Published 27 February 2017
-
Mobilizers
Phoebe Rice
Published 31 January 2017
Escape Artist
Katya Heldwein
Published 19 December 2016
-
Nature’s Confectioner
Jochen Zimmer
Published 29 November 2016
State of Fusion
Jason McLellan
Published 27 October 2016
-
Here Be Dragons
Brian Fox
Published 28 September 2016
SBGrid Assumes Ownership of PyMOLWiki
Published 15 September 2016
-
Pharm Team
Oleg Tsodikov
Published 24 August 2016
Spiro-Gyra
Alejandro Buschiazzo
Published 27 July 2016
-
Turning the DIALS
Nicholas Sauter
Published 29 June 2016
Pipeline Dreams
Bridget Carragher and Clint Potter
Published 26 April 2016
-
U-Store-It
The SBGrid Data Bank provides an affordable and sustainable way to preserve and share structural biology data
Published 28 March 2016
Big Questions, Big Answers
Jennifer Doudna
Published 22 February 2016
-
Not a Structural Biologist
Enrico Di Cera
Published 17 December 2015
Divide and Conquer
Kevin Corbett
Published 19 November 2015
-
Computing Cellular Clockworks
Klaus Schulten
Published 23 October 2015
Trans-Plant
Gang Dong
Published 26 September 2015
-
Keep on Moving
James Berger
Published 23 August 2015
Totally Tubular
Antonina Roll-Mecak
Published 27 July 2015
-
From Disorder, Function
Julie Forman-Kay
Published 29 June 2015
Into Alignment
Geoff Barton
Published 27 May 2015
-
Two Labs, Many Methods
Michael Sattler
Published 28 April 2015
Picture This
Georgios Skiniotis
Published 20 March 2015
-
Intron Intrigue
Navtej Toor
Published 20 February 2015
Cut and Paste
Martin Jinek
Published 28 January 2015
-
Basics and Beyond
Qing Fan
Published 18 December 2014
Bloodletting and Other Studies
Pedro José Barbosa Pereira
Published 25 November 2014
-
Wire Models, Wired
A brief history of UCSF Chimera
Published 29 October 2014
In Search of…New Drugs
Doug Daniels
Published 30 September 2014
-
An Affinity for Affinity…and Corals
John C. Williams
Published 29 August 2014
Pete Meyer, Ph.D.
Research Computing Specialist
Published 22 August 2014
-
Justin O'Connor
Sr. System Administrator
Published 20 August 2014
Carol Herre
Software Release Engineer
Published 15 August 2014
-
Elizabeth Dougherty
Science Writer
Published 13 August 2014
Andrew Morin, Ph.D.
Policy Research Fellow
Published 11 August 2014
-
Jason Key, Ph.D.
Associate Director of Technology and Innovation
Published 8 August 2014
Piotr Sliz, Ph.D.
Principal Investigator, SBGrid
Published 1 August 2014
-
New Kid on the Block
James Chen
Published 29 July 2014
Membrane Master
Tamir Gonen
Published 30 June 2014
-
The Natural Bridge
Piotr Sliz
Published 13 June 2014
Surprise, Surprise
Catherine Drennan
Published 26 April 2014
-
Gone Viral
Olve Peersen
Published 20 March 2014
All Who Wander Are Not Lost
Frank Delaglio
Published 24 February 2014
-
The Raw and the Cooked
Graeme Winter
Published 24 January 2014
Vacc-elerator
Peter Kwong
Published 17 December 2013
-
Structural Storyteller
Karin Reinisch
Published 15 November 2013
The Fixer
Jane Richardson
Published 28 October 2013
-
Inside the Box
Mishtu Dey
Published 17 September 2013
Sensing a Change
Brian Crane
Published 16 August 2013
-
Towards Personalized Oncology
Mark Lemmon
Published 16 July 2013
Brush with Fame
Yizhi Jane Tao
Published 14 June 2013
-
Toxic Avenger
Borden Lacy
Published 21 May 2013
Pushing the Boundaries
Stephen Harrison
Published 22 April 2013
-
Strength in Numbers
Joseph Ho
Published 18 March 2013
One Lab, Many Methods
Wesley Sundquist
Published 12 February 2013
-
Unplanned Pioneer
Tim Stevens
Published 15 January 2013
From Actin to Action
Emil Pai
Published 11 January 2013
-
Unstructured
A Brief History of CCP4
Published 12 December 2012
Stop, Collaborate and Listen
Eleanor Dodson
Published 5 November 2012
-
X-PLORer
Axel Brunger
Published 1 October 2012
Share the Wealth
Zbyszek Otwinowski
Published 22 August 2012
-
Unraveling RNA
Anna Pyle
Published 18 July 2012
Sharper Image
Pawel Penczek and SPARX
Published 4 June 2012
-
Creative Copy Cat
Pamela Bjorkman
Published 25 April 2012
Charm and Diplomacy
Gerard Kleywegt
Published 7 March 2012
-
From Curiosity to Cure
Marc Kvansakul
Published 13 December 2011
The Lure of the Sandbox
Paul Emsley and Coot
Published 15 October 2011
-
Springsteen, Tolkien, Protein
Alwyn Jones and Frodo
Published 17 June 2011
Structures Solved Simply
Paul Adams and Tom Terwilliger on Phenix
Published 2 June 2011
-
Escape from the Darkroom
Wolfgang Kabsch and XDS
Published 19 May 2011
Playing the Odds
Randy Read and Phaser
Published 19 May 2011
-
Crystallography for Kids
Lynne Howell
Published 17 May 2011
Better, Faster, Stronger, More
Victor Lamzin and ARP/wARP
Published 17 May 2011